Consider the cells of our bodies as miniature soldiers, continuously confronting potential threats from injury and disease. To equip these cellular soldiers to better withstand these threats, researchers in the Bozhi Tian research group have pioneered a novel method to enhance cellular stress tolerance through precise optoelectronic control of cellular processes. The study, published April 15th in Nature Nanotechnology, represents an innovative convergence between bioelectronics and intracellular biomolecular condensates, a rapidly evolving area of cell biology.
“This is important because in our work, this is really the first time we’ve integrated those two rapidly emerging fields together,” says Professor Tian.
To help cells better withstand threats, the group focused on stress granules. These are dynamic liquid structures that assemble inside cells whenever they face challenging conditions. The group developed a new technique that uses light-activated gold-modified silicon nanowires to produce small amounts of hydrogen peroxide, an important signaling molecule. By doing this, they essentially prepare the stress granules, making the cells more resilient to stronger, subsequent stresses.
This underlying principle of pre-conditioning cells mirrors how traditional vaccines work, says Jing Zhang, a Post-Doc scholar with the Tian group and first author of the study.
“The role of a vaccine is to enhance the body's tolerance level to an even larger virus threat that may appear later on. You can think of the role for those stress granular in this way,” says Zhang.
The inspiration for this method originates from the natural world, says Zhang; specifically from defense mechanisms observed in plants. Plants utilize a specialized enzyme within their photosynthetic machinery, known as a photosystem II. These enzymes produce highly reactive molecules called reactive oxygen species (ROS) when exposed to intense light. This production helps the plant adapt and, according to Zhang, “become more robust and tolerant to larger oxidative stressors or light intensity.”
To translate this biological strategy into a controllable system, the researchers developed their method by utilizing a carefully engineered nanocatalyst composed of gold-modified nanoporous silicon, a material chosen for its ability to produce low levels of hydrogen peroxide without depleting vital oxygen.
“We don't want to use a lot of oxygen because of its importance in maintaining heart function,” says Zhang.
Luckily, the gold-modified silicon system developed by the team was successful in creating hydrogen peroxide that doesn't consume the body's oxygen. Additionally, this photocatalytic approach was shown to be effective in a lab-maintained heart model that simulated injury from blocked and restored blood flow.
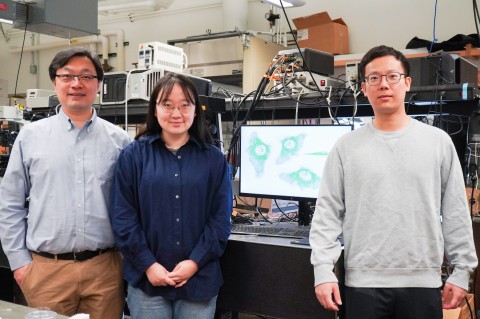
Tian and Zhang believe their approach can directly address any medical issue involving ischemia, a condition where blood flow is restricted in the body.
For instance, during a heart attack, the temporary interruption of blood flow (ischemia) and its subsequent restoration (reperfusion) lead to the generation of damaging molecules. This process of ischemia-reperfusion injury is not unique to the heart; it also underlies tissue damage in conditions such as pressure ulcers and ischemic stroke.
Pengju Li, a graduate student with the Tian lab who also contributed to the study, remarked, “In our previous Nature paper, we used fast electrical modulation to control heartbeats. This time, we offer a subtler, longer-lasting approach to boost heart resilience. It marks another milestone for the Tian Lab and the field, grounded in subcellular fundamentals.”
Looking beyond immediate applications in heart health, the researchers anticipate that precisely controlling stress granule formation with their technology could enhance cellular resilience and offer new therapeutic avenues across a wide range of medical fields, including accelerated tissue regeneration, providing a powerful new tool for modulating cellular responses to disease and injury.